Legal
How to Find Best Mesothelioma Lawyers
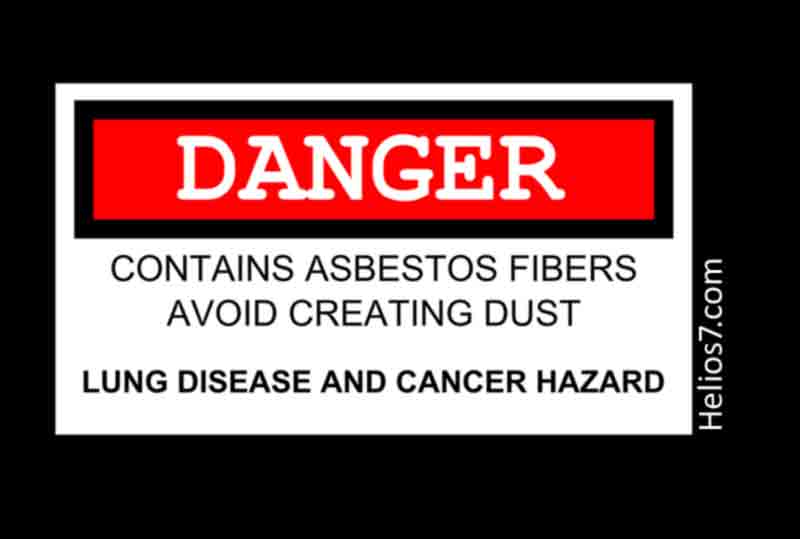
Selecting a mesothelioma attorney at law is an important step. If you are a victim of mesothelioma then you must get in touch with best mesothelioma lawyer who will help you receive justice and compensation which you truly deserve.
Mesothelioma Lawyers Advice provides few of the top mesothelioma legal resources and asbestos law information. You can find best mesothelioma hospitals, lawyers, attorneys, law firms, treatment centers and many many more.
If you wish to include your Mesothelioma Law Firm in this top mesothelioma directory then do send inclusion request 6lawyermarketing[at]gmail.com